As the world prepares for the 26th UN Climate Conference of Parties (COP26), we review some of the key facts of global warming and climate change. We will start this review with the physics behind it all; after all, where would we be without quantum mechanics?
Part 1 - The Physics of Global Warming
All work is powered by energy conversions. On Earth, the dominant energy conversion is the transformation of higher energy light particles from the Sun into lower energy light particles through physical and chemical processes.
The physical conversion of sunlight drives the ocean currents, wind patterns, and the water cycle. The chemical conversion of sunlight drives atmospheric and oceanic chemistry, and enables photosynthesis, the primary energy source for life on Earth. Photosynthesis uses sunlight to convert water and carbon dioxide into glucose and oxygen. The solar energy stored in the chemical bonds of glucose is then used by organisms to move and grow and is ultimately dissipates as heat. Organisms with effective photosynthesis are able to store about 1% of the annual solar energy they receive.
The next great energy conversion on Earth is the radioactive decay of elements such as Uranium and Thorium in the molten mantle. Together with other physical processes, this conversion creates the continents and renews the carbon dioxide in the atmosphere.
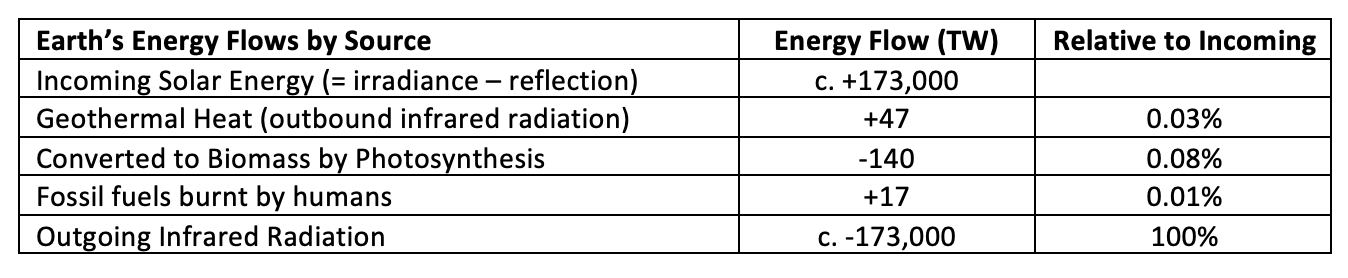
Figure 1 - Earth's Energy Budget (humanity inserted for scale)
The energy flows into and away from any planet or moon are in radiative equilibrium; over geologic time scales the difference between energy inflows and outflows average to zero and the long-term average temperature remains within a narrow band. In the absence of an atmosphere, the radiative equilibrium is determined by a few (mostly) independent factors, such as rock properties and exposure time, and the resulting long-term average surface temperature varies only very little, if at all.
Earth’s atmosphere and hydrosphere on the other hand introduce many (mostly) interdependent factors due to the various interlinked internal heat transport processes (e.g., wind, ocean currents, water cycle), ever-changing reflectivity (e.g., clouds, snow cover), and the differing absorption spectra of the various atmospheric components, all of which means that Earth’s long-term average surface temperature may fluctuate considerably, sometimes even rapidly compared to the geological time scales.
The atmosphere acts as an insulator around Earth’s surface; it absorbs about 30% of the incoming solar radiation and about 80% of the outgoing infrared radiation. Figure 2 shows how this works. The red line in the top shows the wavelength distribution and relative intensity of the sunlight that reaches the Earth’s outer atmosphere, and the solid red shape shows the portion that makes it directly to the Earth’s surface. The blue line and shape show the distribution of the infrared light departing from the Earth’s surface and the portion that makes it directly into space.
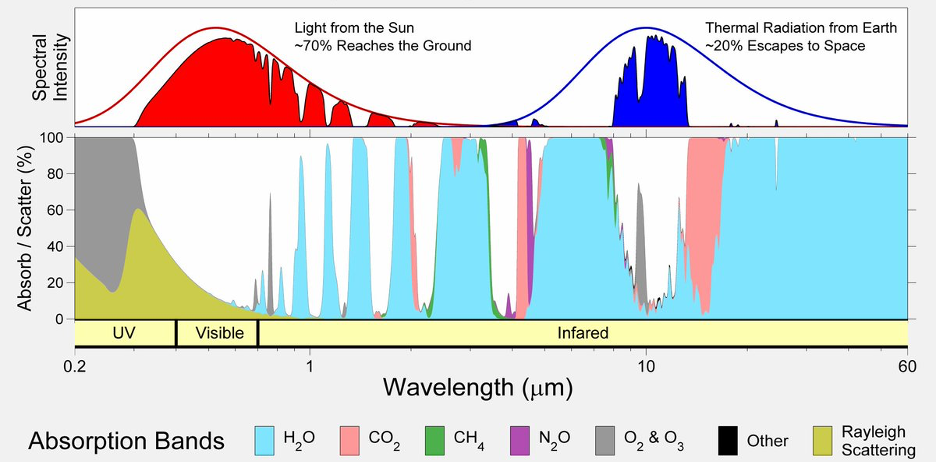
Figure 2 – Absorption Spectra of Greenhouse Gases vs Incoming and Outgoing Radiation (HiTran Data)
Light particles (photons) in the ultraviolet (UV) range carry enough energy to excite a molecule’s electron from its stable ground state into a higher orbital shell. Typically, the excited electron decays back into its ground state within a few nanoseconds by emitting the photon. The net effect of this kind of absorption event is a random redirection (scattering) of the incoming photon. If there is an abundance of absorbing molecules, there will be many random scattering events, the net effect of which will be that nearly all incoming photons are scattered back into space.
Deep UV light from the Sun (wavelength ≤0.2µm) is scattered back into space by abundant nitrogen and oxygen gas molecules and does not reach the Earth's surface at all. The ozone layer scatters back ±98% of all incoming medium UV light (0.200-0.315µm). Near UV light (the kind that causes common sunburn) is not absorbed by the atmosphere and is only affected by a physical effect known as Rayleigh scattering which sends ±30% back into space.
Photons in the infrared (IR) range do not carry enough energy to excite electrons, but they can cause changes to the vibrations and rotations of larger and/or asymmetric molecules such as water, carbon dioxide, and methane. Water has a great many different vibrational modes which means it can absorb a wide range of photons in the IR range.
Figure 2 shows the absorption spectra of water vapor, carbon dioxide, methane, and nitrous oxide in light blue, light coral, lime green, and magenta respectively. Each these gases close a part of the window for direct transmission of photons in the IR range. Like UV photons, absorbed IR photons eventually find their way out into outer space, after many random scattering events.
If the concentration of greenhouse gases increases, the amount of IR photon scattering in the absorption bands increases. This means that IR photons spend more time bouncing around in the atmosphere before flying off into space. The increased residence time of the photons increases the energy density of the atmosphere, or temperature. This is called “the greenhouse effect” and it boosts the Earth’s average surface temperature by around 33 degrees Celsius. Without the temperature boost from the greenhouse effect, Earth's average surface temperature would be around -18°C, meaning that liquid water would only exist in the vicinity of volcanic activity and that life as we know it could not have evolved.
The amount of water vapor in the atmosphere is determined by the water cycle, which is driven by the average surface temperature. The presence of water vapor on its own would not be enough to sustain a greenhouse effect; over geologic time, a “water vapor only” Earth would cool down to the radiative balance temperature of -18°C.
Earth's greenhouse effect is driven and maintained by the quantities of carbon dioxide pushed into the atmosphere by volcanic activity and burning biomass, and by methane emissions from biomass. Since atmospheric carbon dioxide and methane have a half-life of 100 and 9 years respectively, constant renewal of these gases is necessary to maintain the status quo. Conversely, any significant disturbance of these renewal processes will lead to changes to the temperature boost by the greenhouse effect.
On the scale of the global energy inflows and outflows, the sum of human activity seems like a mere rounding error. However, it turns out that the wholesale burning of fossilized biomass can and does impact the average global surface temperature in the near term.